Theory of Large Loop Antennas
last updated 2 December 2023.
While 1 wavelength loops radiate broadside to the plane of the antennas, larger sizes tend to radiate more off the edges, and may have a broadside null. With larger loops (in terms of wavelengths), the patterns can have multiple lobes and nulls. We most often encounter this when we use a single loop antenna on multiple bands.
Note that, while the loops I use for illustrations will be regular polygons, in practice loops can be a wide variety of shapes and sizes. The radiation pattern and feedpoint impedance will change in the process, but, in most cases the loop will still work similar to a polygon of the same circumference (as long as the area enclosed by the loop is not too small). So don’t consider these examples as the only options. However, there is a limit to how many different examples I can include in one article, and these should help you get a general idea of the effects of shape and circumference.
Let’s start with a simple square loop in free space, fed in the middle of one side, and see how the radiation pattern and other characteristics vary as we change the frequency (or change the length in wavelengths, which is the same thing).
Square loops in free space
Half wave loop
Let’s start small: a loop about 1/2 wavelength in perimeter (typically closer to 0.52 wavelengths at resonance). If we analyze the current distribution, there is only one current maximum, at the point opposite the feedpoint, and the feedpoint is at a high impedance point.
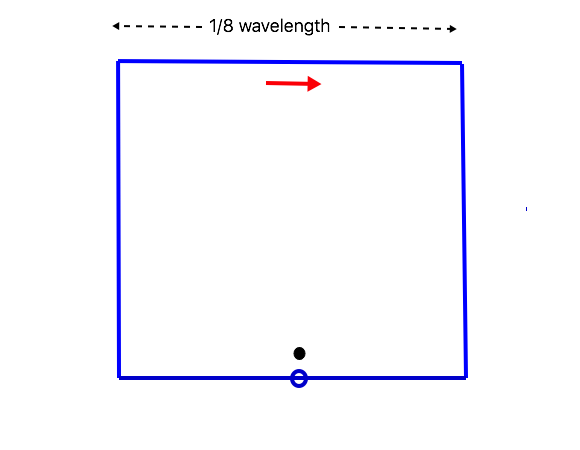
Because there is only one current maximum, the resulting pattern is more like a dipole, but the radiation from the folded ends makes the pattern more omnidirectional: the difference in gain is no more than about 4 dB for any two directions. However, the feedpoint impedance is very high – tens of thousands of ohms according to modeling software, making matching more difficult. If the loop is opened at the point opposite the feedpoint, it becomes a bent dipole rather than a loop, and the feedpoint impedance is much more manageable (around 10 – 15 ohms). This shape is the basis of “Halo” antennas for VHF, and the “cobweb” antenna at HF.
While my 1956 ARRL Antenna Book discusses the half wave loop, I can’t think of any practical antennas that actually make use of it as a loop, rather than a bent dipole.
Full wave loop
This is probably the most common size of loop, and it has its own theory page that goes into much more detail of different shapes and feedpoint impedances.
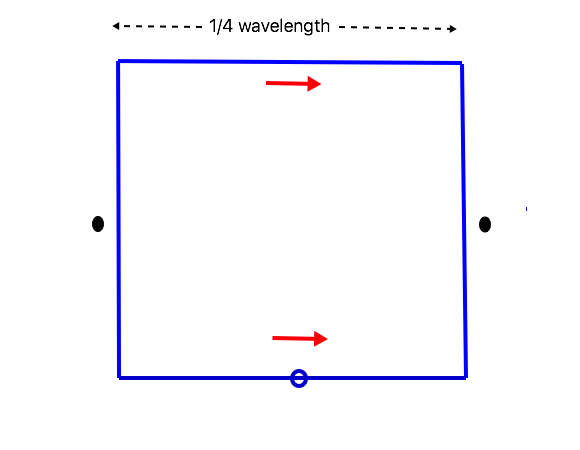
For the purpose of this discussion, note that it has two current maxima, with the currents in phase for maximum radiation broadside to the plane of the loop. It has a manageable feedpoint impedance (around 120 ohms), and gain is slightly higher than a dipole.
2 wave loop
The 2-wavelength loop introduces a significant change in radiation pattern. Let’s look at the current distribution:
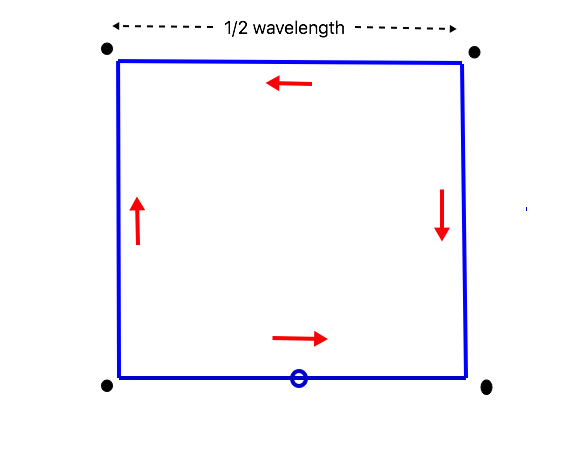
When viewed broadside to plane of the loop (the reader’s view of the page), each current arrow has another that is equal but in the opposite direction. That signifies a null broadside to the antenna. Now consider the radiation to the right of the drawing: the top and bottom arrows don’t contribute (since they are at right angles), and the right and left arrows are out of phase. But the sides are spaced 1/2 wavelength apart, and by the time the radiation from the left side reaches the right side, the current in the antenna has shifted phase by 180 degrees, so the radiation from the two sides are in phase off the sides of the loop.
On the other hand, looking at the antenna from the upper right corner, the currents in the bottom and left wire will cancel each other, as will the currents in the top and right wires. (The currents in each pair are equidistant from the viewer, so we don’t need to adjust for transit time.) As a result we have nulls off the corners, and a pattern in the plane of the loop that looks like this:
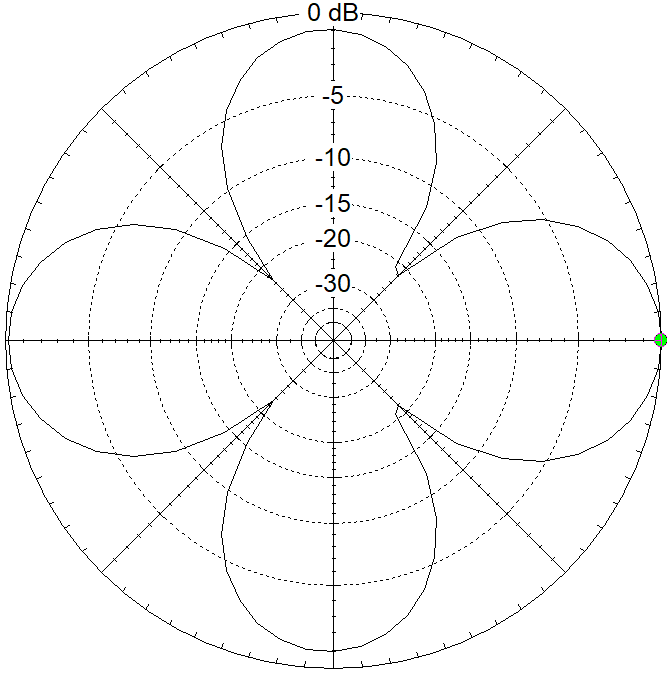
Given that there is a null broadside to the loop, and useful lobes off the edges, the most effective way to use this as an antenna is to install it horizontally above ground. Vertical plane loops tend to waste more radiation at high angles when they are 2 wavelengths or longer, which limits their application as multiband antennas.
The feedpoint impedance of the 2 wavelength loop is around 260 ohms at the resonant frequency (which is a bit lower than twice the fundamental). Again, this is relatively convenient to match.
As we increase the loop length (or the frequency), we tend to see more lobes and nulls in the pattern. The exact locations depend on the shape of the loop and the feedpoint location, as they are dependent on both the locations of the current maxima and the spacing between them.
When used as a multiband antenna, a horizontal loop will have maximum radiation straight up (depending on height) on the fundamental where it is 1 wavelength, making it useful for NVIS paths, and progressively lower angles of radiation on the higher bands where that is useful for DX. As a result, horizontal loops for 40m, 80m, or 160m are popular antennas for multiband operation, in spite of the various lobes and nulls in the pattern.
Non-resonant lengths will also work: the pattern might not be as symmetric, and the feedpoint impedance may not be as easy to match (and can be in the thousands of ohms when the loop is an odd multiple of 1/2 wavelengths) but the antenna can still be quite useful. For example, I have installed a loop under the eaves of a house where the length was fixed by the size of the building. With a balanced feedline to a tuner it worked all HF bands, even if the patterns didn’t exactly match the ones given here.
Now that we understand why larger loops are best when installed horizontally, let’s add the ground back under it and look at some typical patterns.
Horizontal loop antennas over ground
The following plots are for horizontal loops resonant at 3.55 MHz installed 10m (33 feet) above the ground. Patterns are given for square and triangular loops, fed in a corner or in the middle of one side. The purpose here is to look at general trends and differences, rather than precise patterns. In each case, the azimuth plot is oriented so that the antenna feedpoint is at the bottom. Azimuth patterns are taken at the angle of maximum radiation, or at 45 degrees elevation, whichever is lower. In practice, elevation angles will vary with height of the loop.
80m patterns (full wave loop)
There is little practical difference among the azimuth radiation patterns when the loop is one wavelength.
There is so little variation that I haven’t even marked which plot is which. Maximum radiation is straight up in all cases, although these plots are at 45 degrees. This works well for NVIS links. Maximum radiation is in the direction of the feedpoint, but not by enough to notice: it is pretty much omnidirectional at high radiation angles.
40m patterns (2 wavelengths)
As we saw previously, the vertical radiation pattern of a square loop fed in the middle of one side has an overhead null, making it less useful for local NVIS use. (The Bow-Tie Loop was developed to work around this limitation.) Let’s start by looking at the vertical radiation patterns of each of our 4 loops:
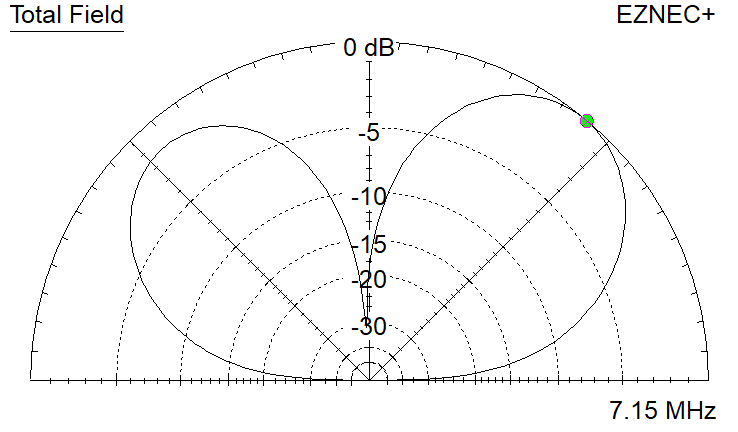
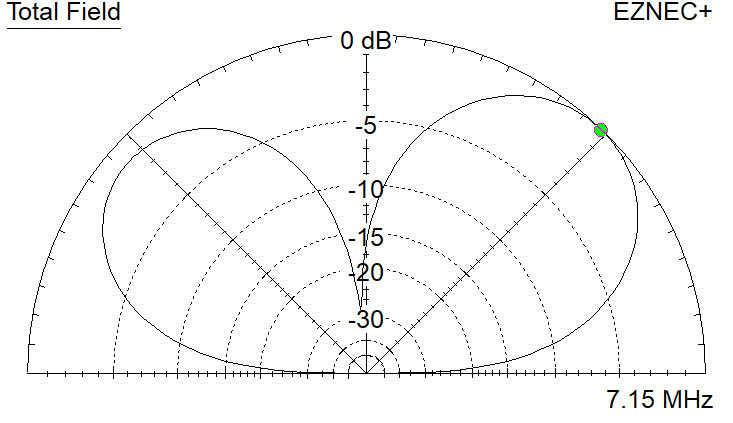
The square loop fed in one corner (left) or one side (right) has an overhead null, and maximum radiation in the direction opposite the feedpoint position. Radiation is maximum at a bit over 45 degrees.
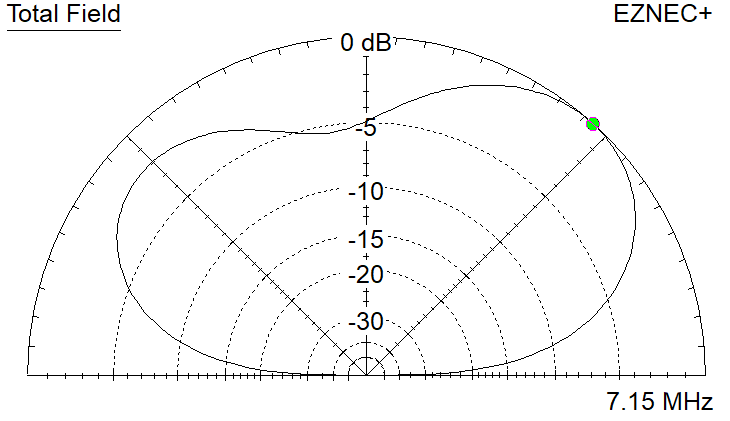
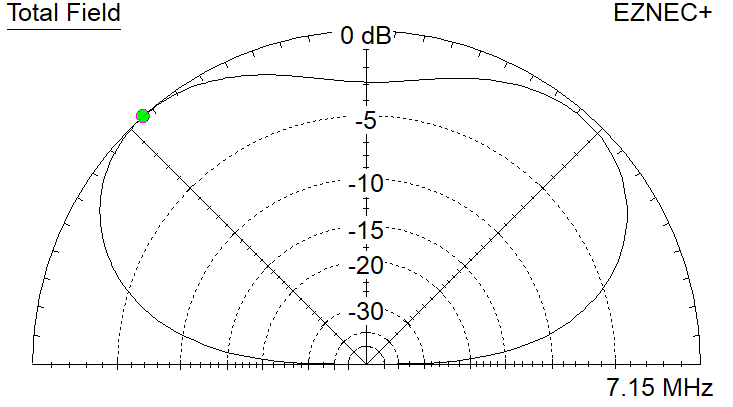
The triangle loops don’t have as much of an overhead null. Maximum radiation is opposite the feedpoint when fed in one corner (left), but slightly in the direction of the feedpoint when fed in the middle of one side (right).
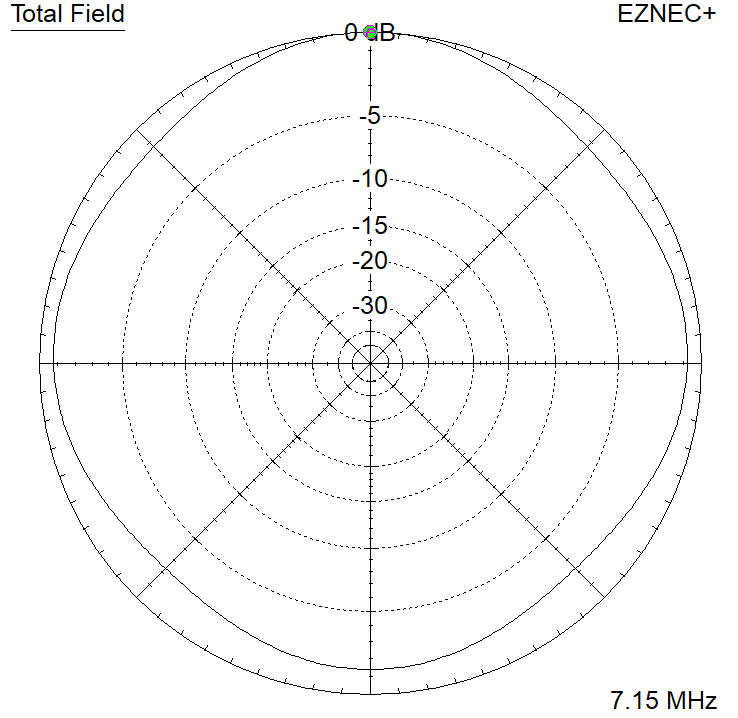
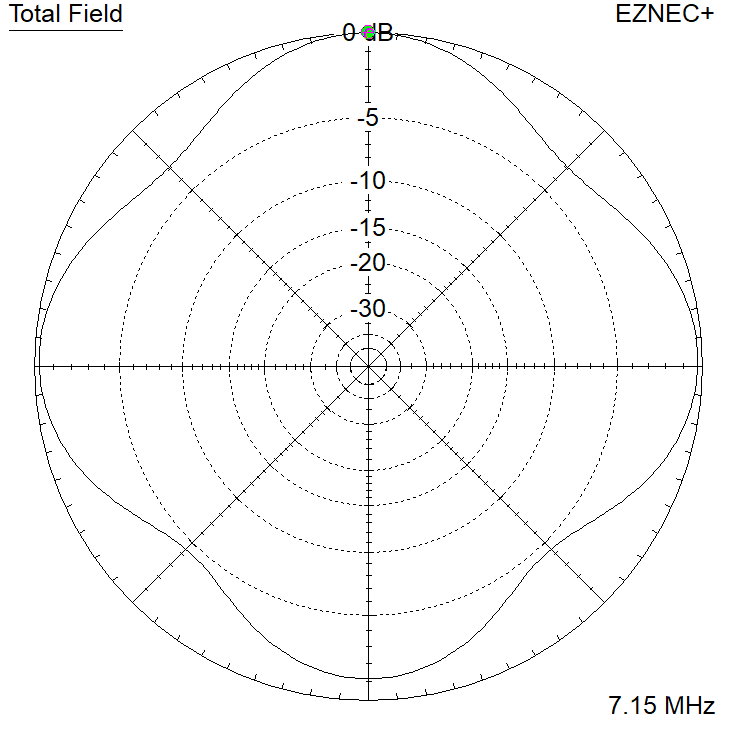
The azimuth pattern on 40m shows more of a lobe structure when fed in a side (right) than in a corner (left), but generally the differences are small.
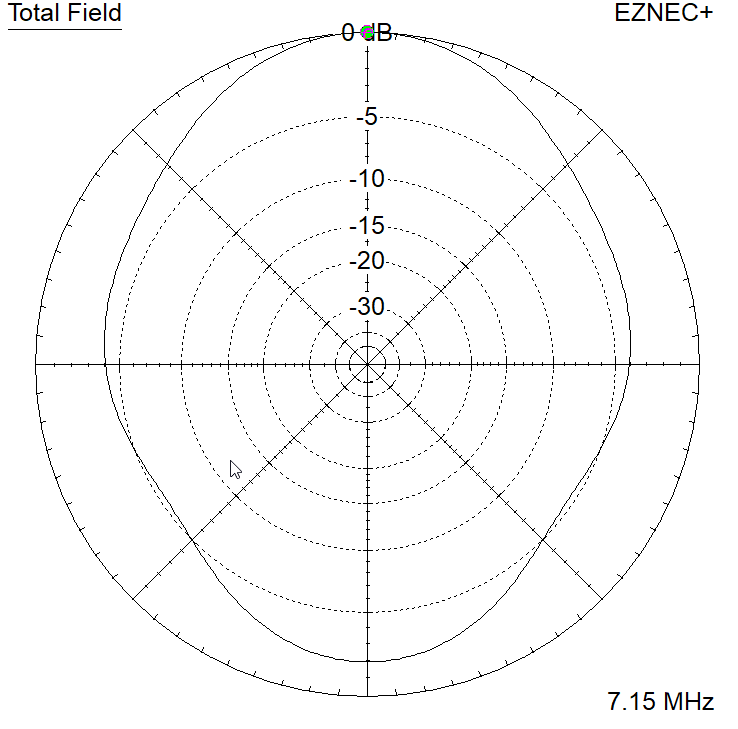
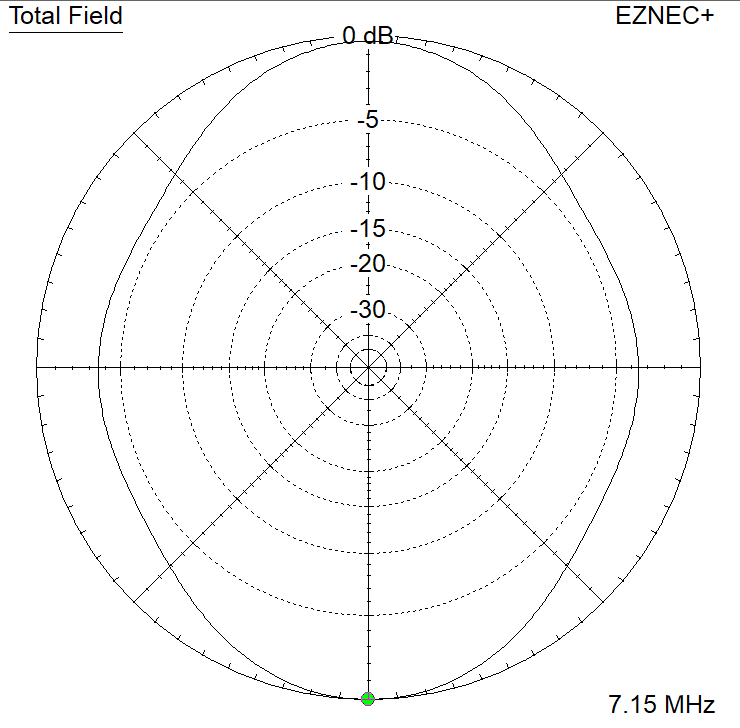
The triangular loops are more bidirectional on 40m, although the differences are still small. Again, maximum radiation is reversed between the two (always off a flat side of the loop). The maximum gain of the triangular loops is up to 1 dB greater than the square loops, due to the more bidirectional pattern.
30m patterns (close to 3 wavelengths)
The 30m patterns show much more variation. They are plotted for 10.1 MHz, although the loops tend to be resonant around 10.7 MHz. Because the differences are greater among them, I will group the plots for each antenna together. While I’ll show both azimuth and elevation plots, the actual patterns for these and the higher frequencies are more complex, and two plots alone won’t show all the detail.
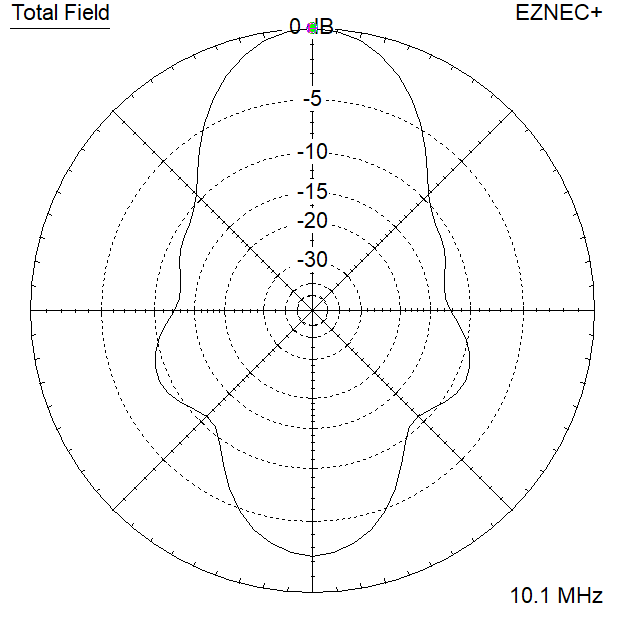
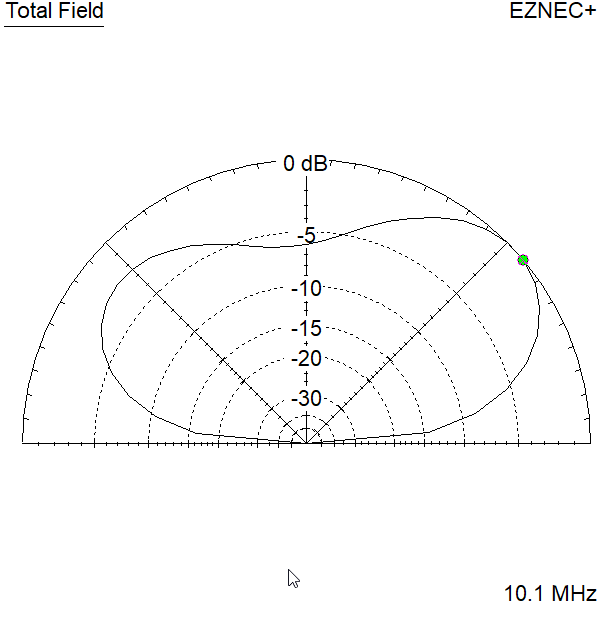
The square fed in one corner shows a strongly bidirectional pattern. As is typical, maximum radiation is opposite the feedpoint.
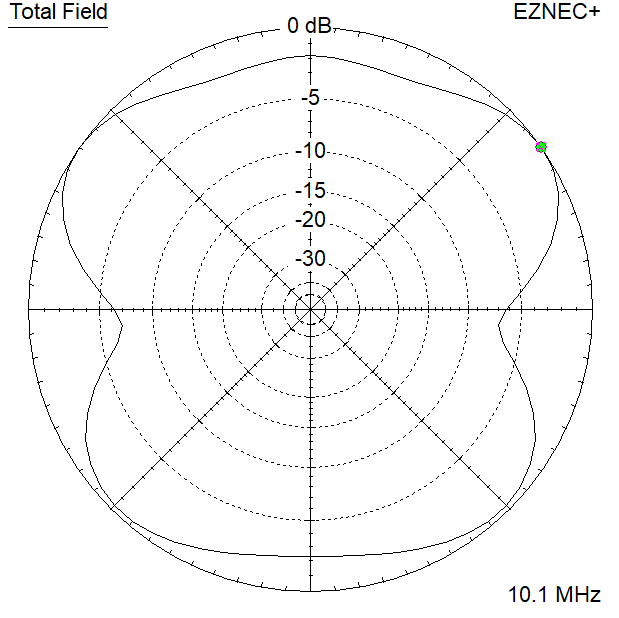
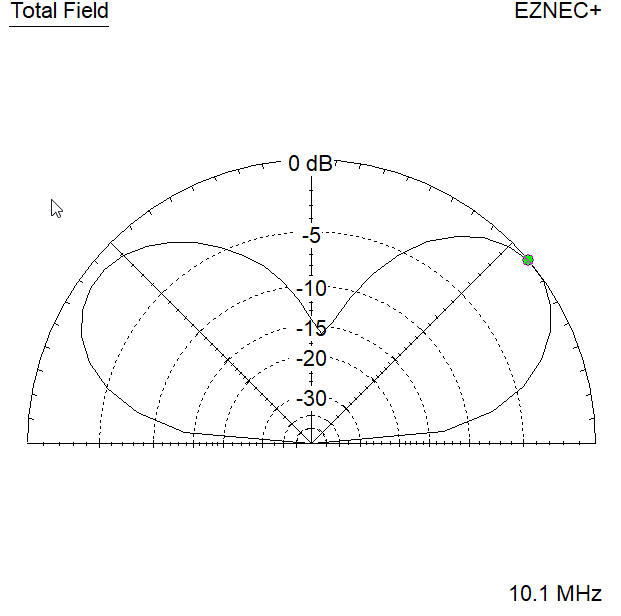
On 30m the square fed in the middle of one side shows a 5-lobed pattern that is generally omnidirectional. The gain doesn’t exceed that of a dipole in any direction, but the nulls aren’t as deep.
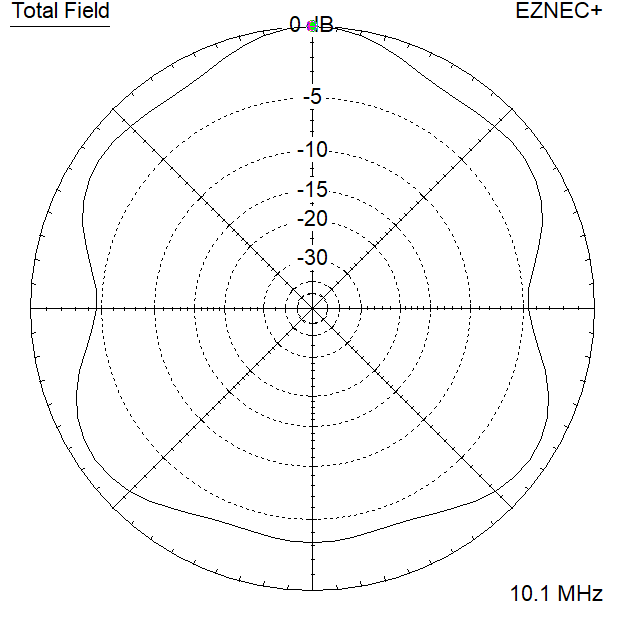
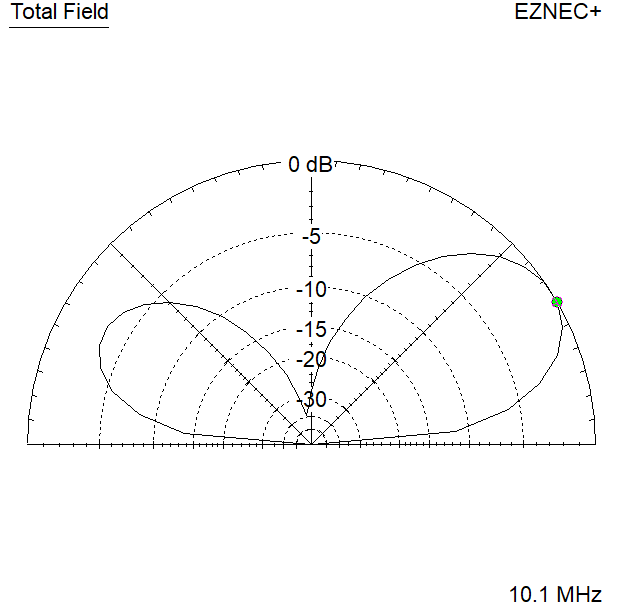
On 30m the triangle fed in one corner resembles the square fed in one side, with a generally onmidirectional pattern.
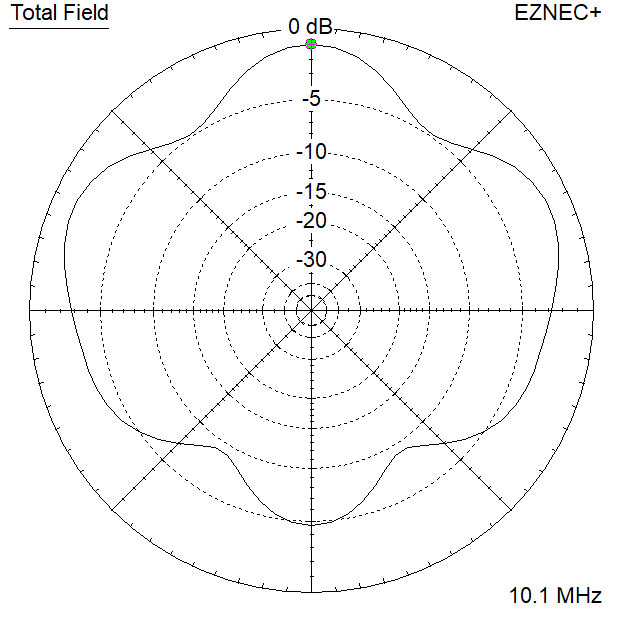
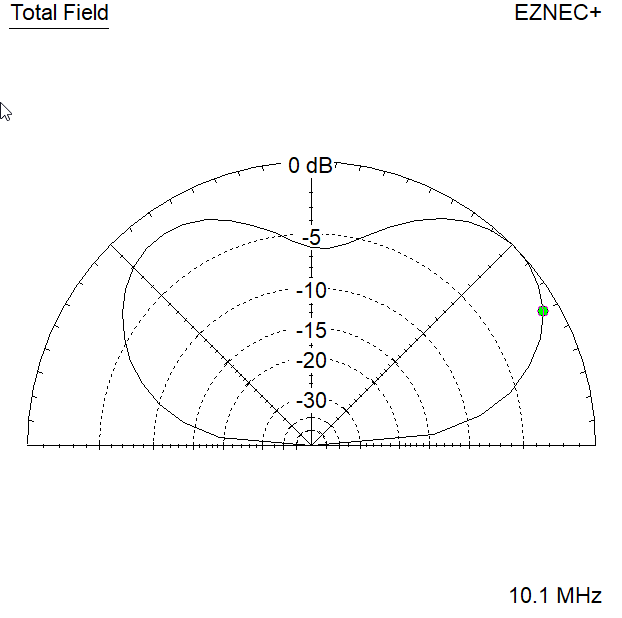
On 30m the triangle fed in one side has maximum radiation opposite the feedpoint, but it is at a higher angle than the other loops. The side “wings” in the pattern have a lower maximum, but peak at a lower angle.
20m patterns (4 wavelengths)
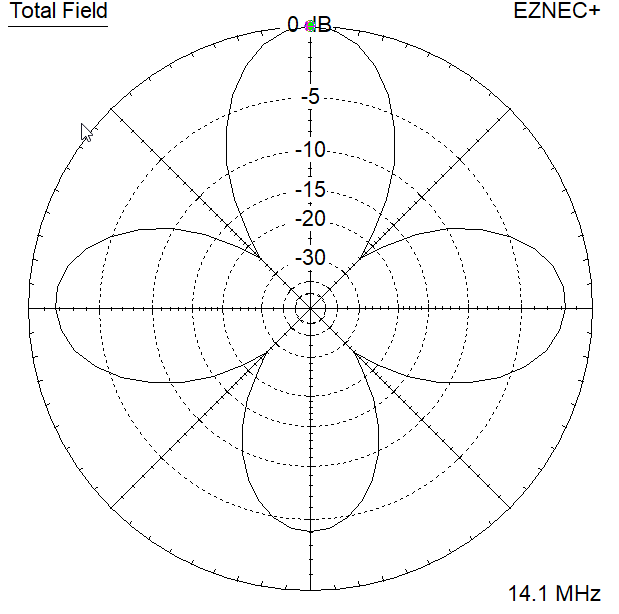
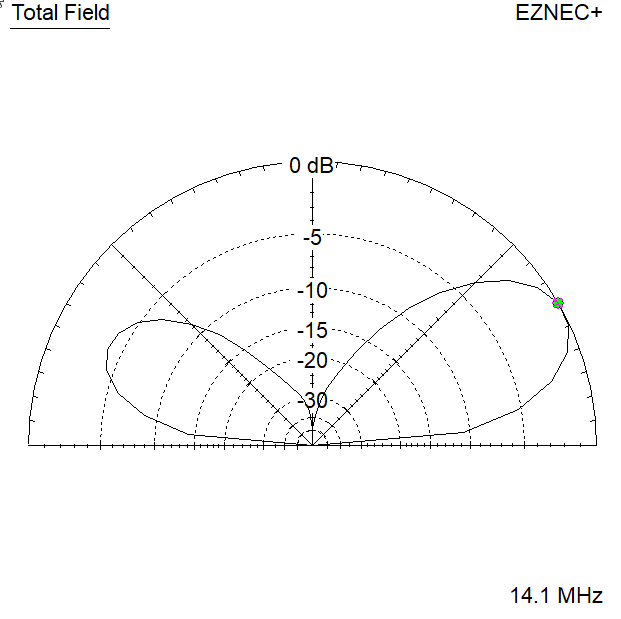
On 20m the square loop fed in one corner has a very clean 4-lobed pattern, at least in a model where the geometry can be precisely controlled. The sharp lobes and nulls might not survive minor variations in dimensions when strung between trees.
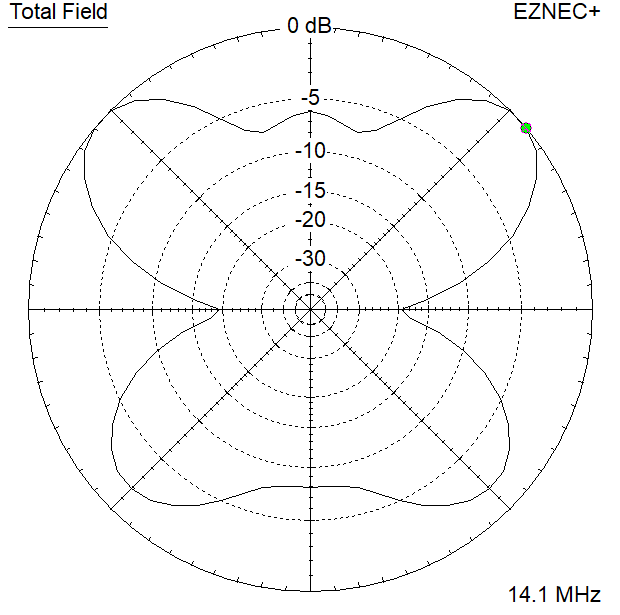
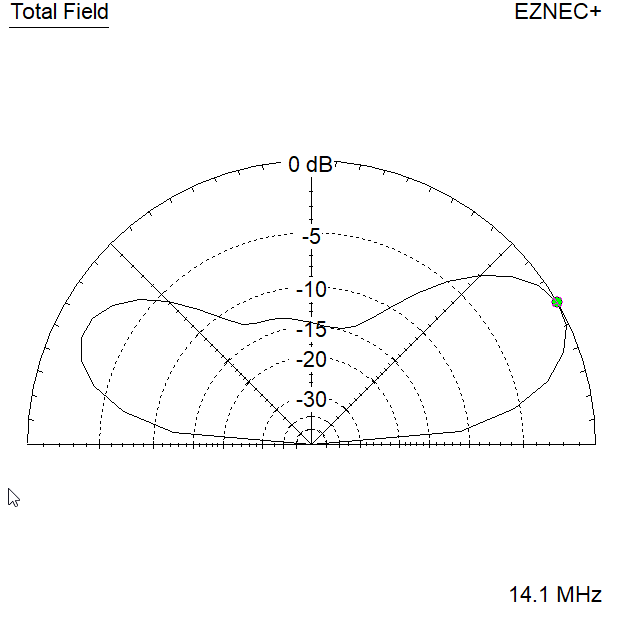
The square loop fed in one side appears to have a very different pattern. However, in both cases, radiation is maximum off the corners of the loop rather than relative to the feedpoint.
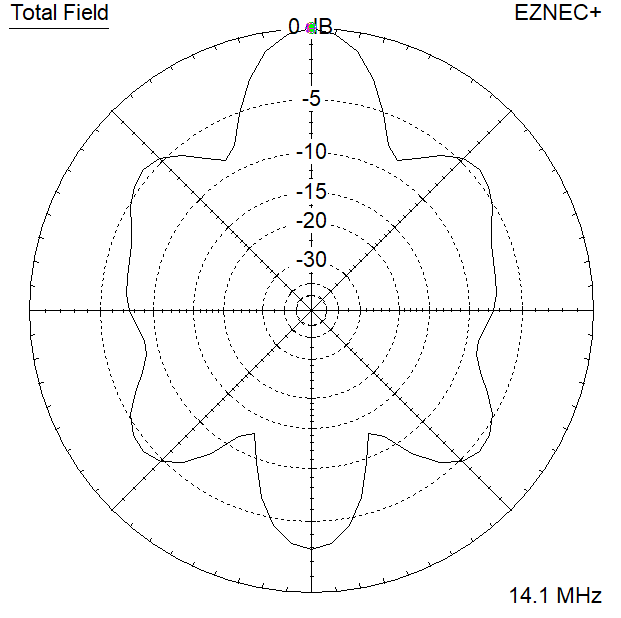
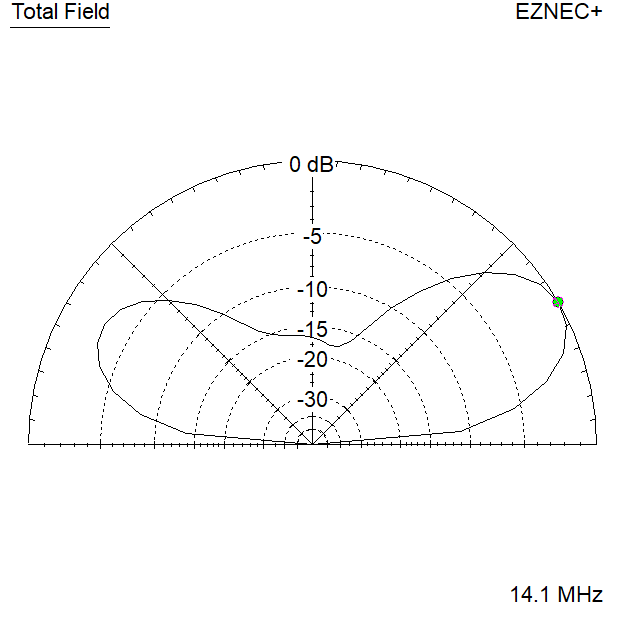
The triangular loop fed in one corner has a more bidirectional pattern.
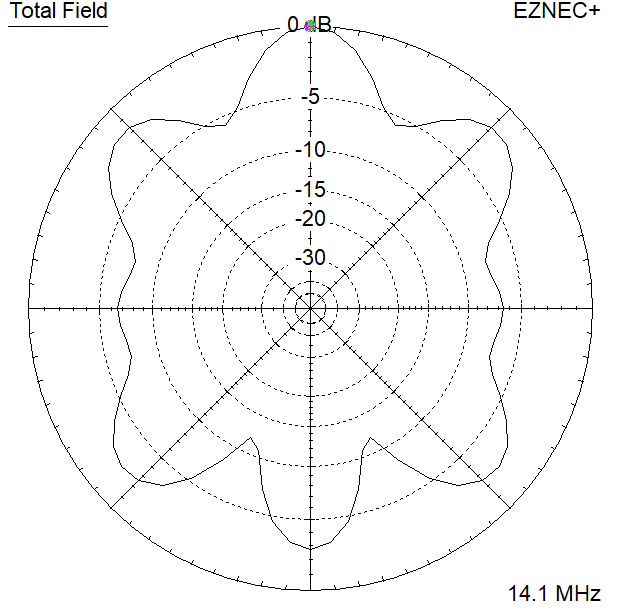
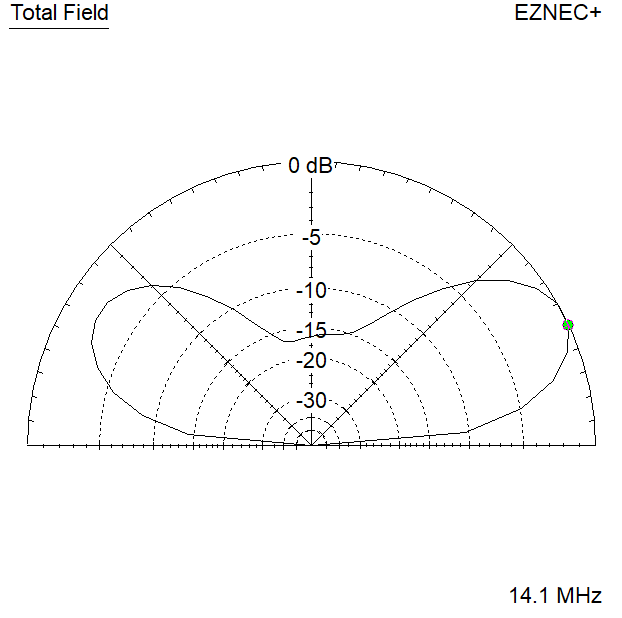
The triangle fed in one side is very similar to the corner feed in this case.
15m patterns (6 wavelengths)
On 15m the antenna is more than 1/2 wavelength above ground, and we start to see minor vertical lobes, in addition to the expected increase in the number of azimuth lobes.
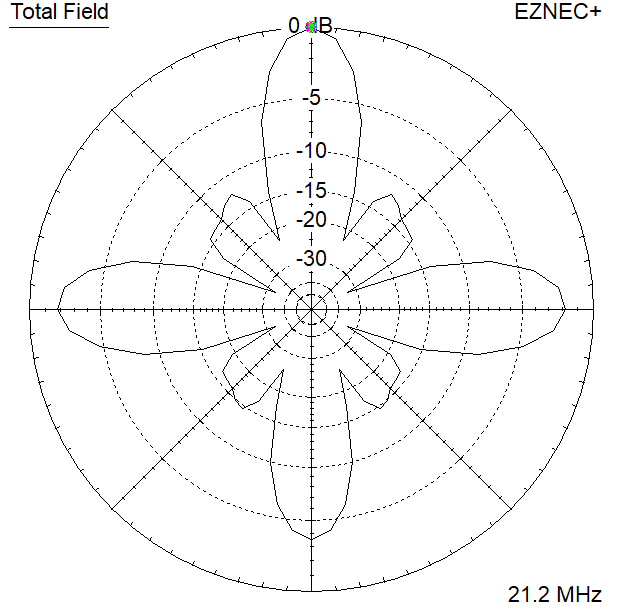
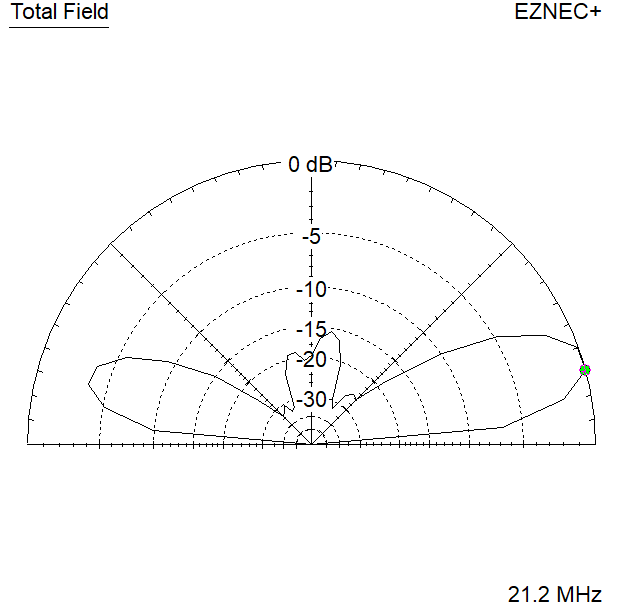
The 15m pattern shows 4 very narrow major lobes with significant gain over a dipole (about 5 dB). The beamwidth of these lobes is only about 20 degrees, requiring careful aiming to make use of them for a specific path. Again, this pattern shows the careful dimensions set up in the modeling software that cause cancellation and reinforcement of lobes due to spacing: less precise dimensions more typical of actual construction may give a different result.
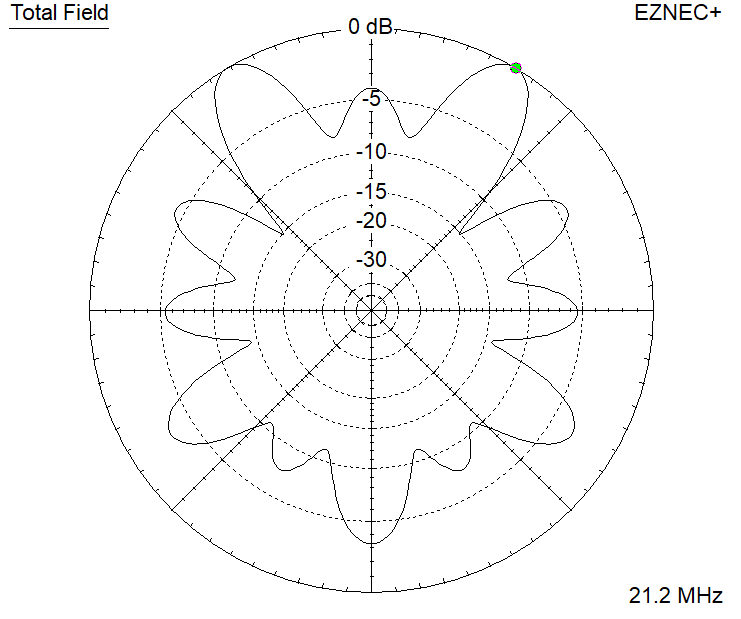
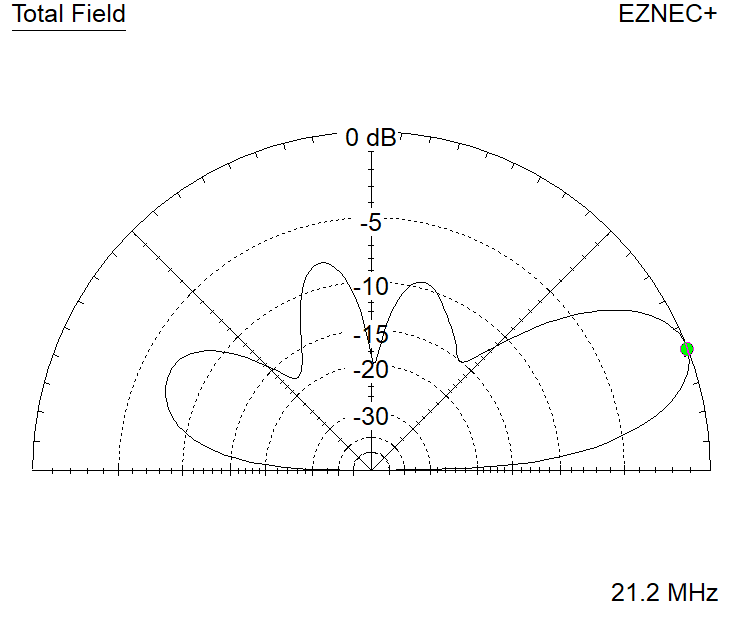
The square loop fed in one side shows what happens when the radiation from the various wires don’t exactly align. The major lobes are about 3 dB better than a dipole, and the large number of lobes and shallower nulls make the pattern more omnidirectional.
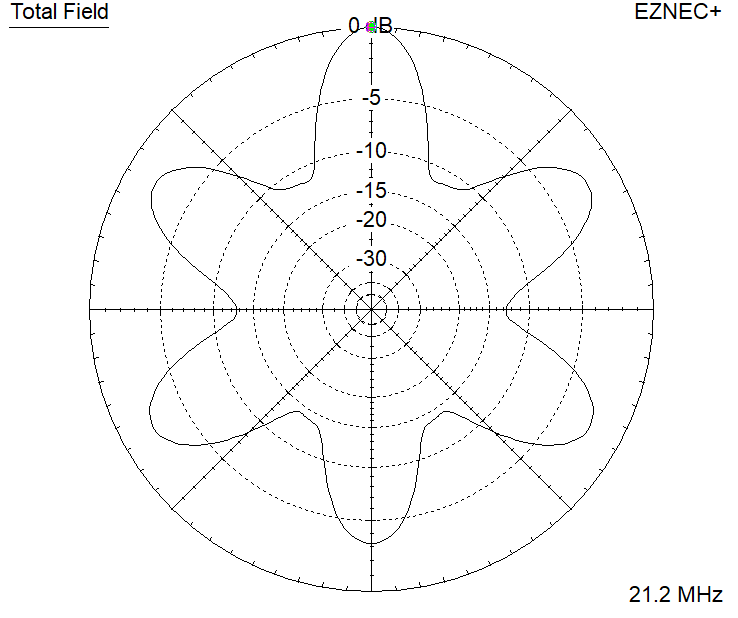
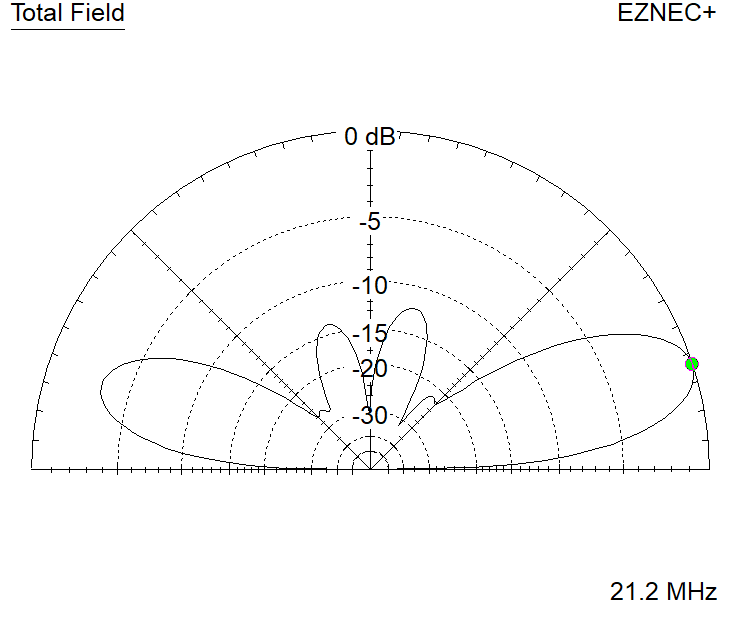
On 15m the triangle fed in one corner has precisely 2 wavelengths per leg, and creates a clean 6-lobed pattern, with the gain in each lobe slightly better than a dipole.
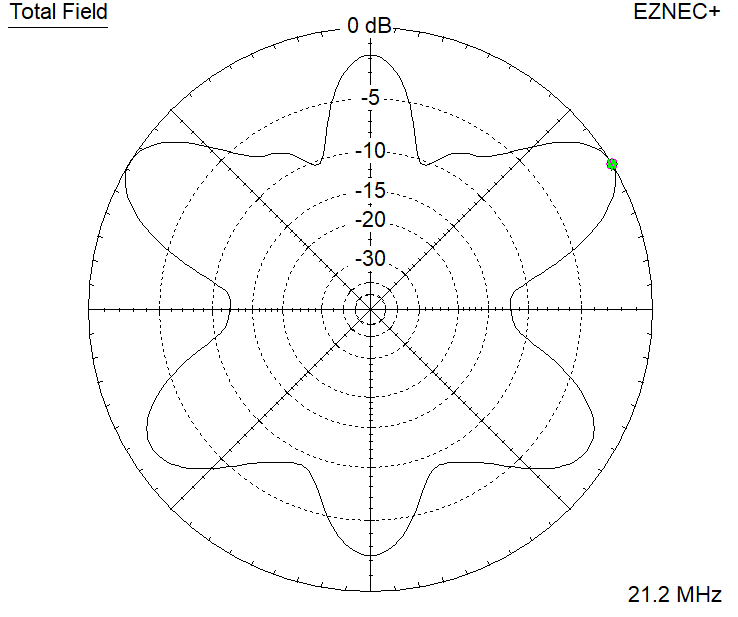
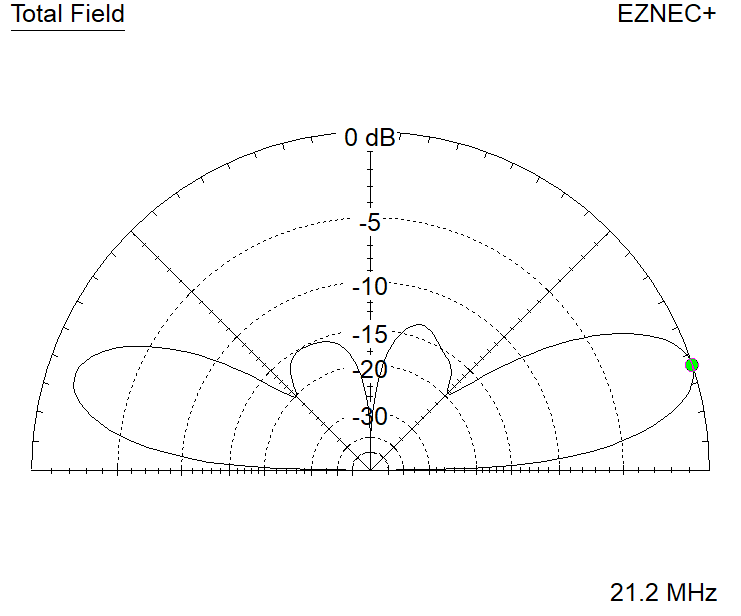
The triangle fed in one side has a similar pattern, but not as symmetric.
10m patterns (8 wavelengths)
On 10m the patterns break up into many more lobes, each being narrower. This may provide contacts in many directions, but not necessarily to any specific station.
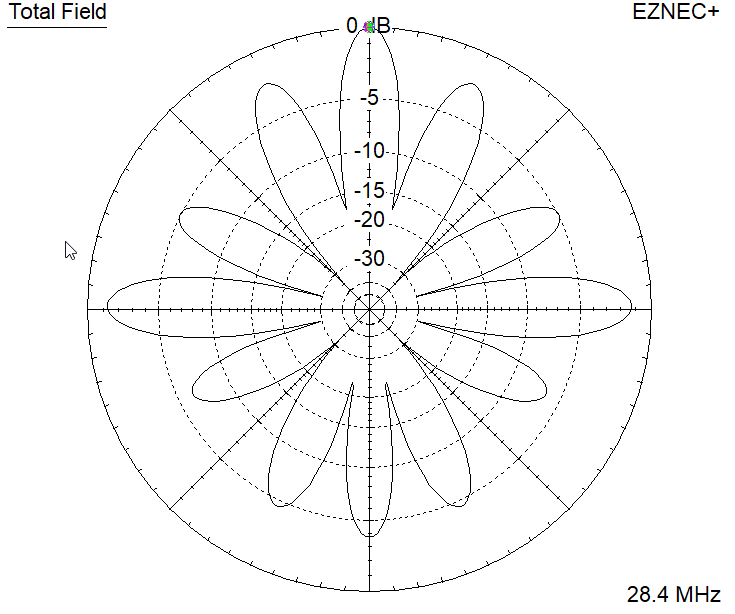
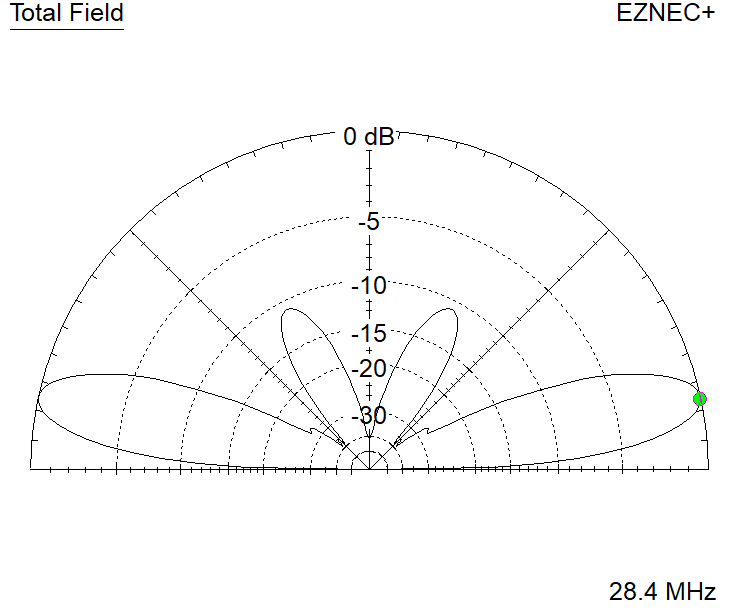
The square fed in one corner still shows maximum radiation away from the feedpoint, now with 12 narrow lobes in the pattern. There are more high angle lobes in other directions not shown on this elevation plot.
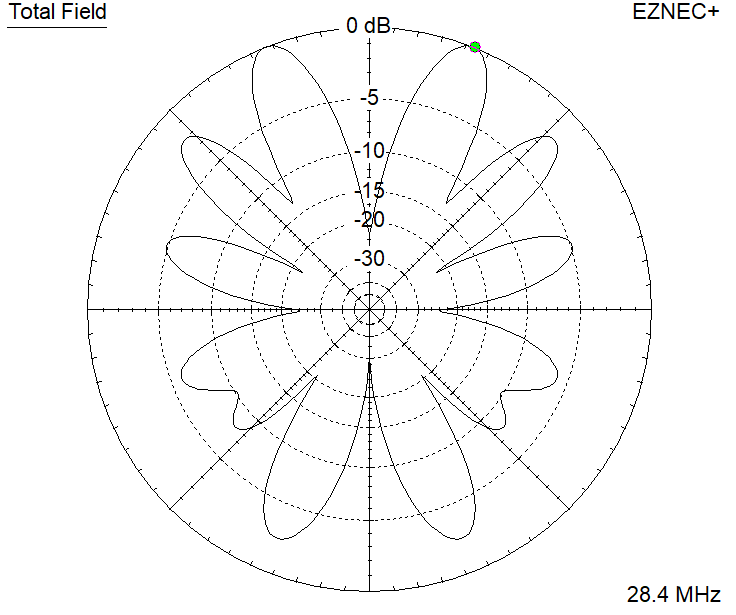
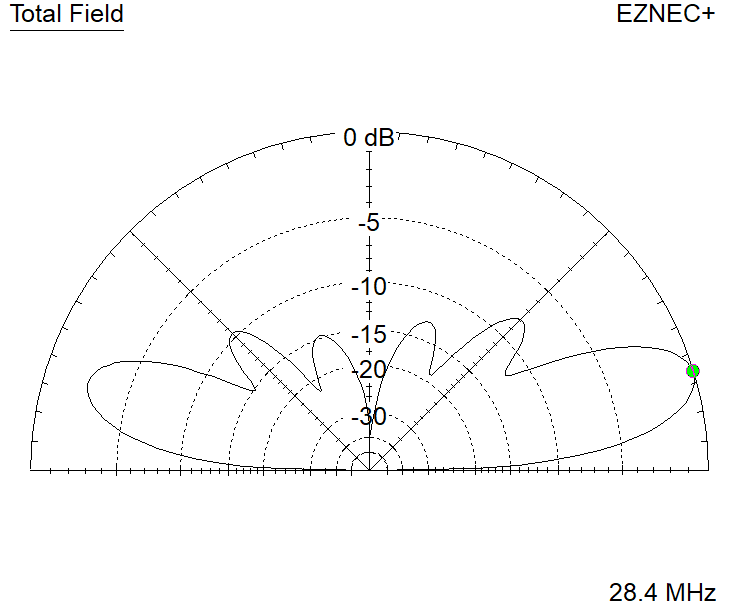
The square loop fed in one side also has 12 lobes, but they are not as evenly distributed around the compass, and there is a null in both directions in a line through the feedpoint.
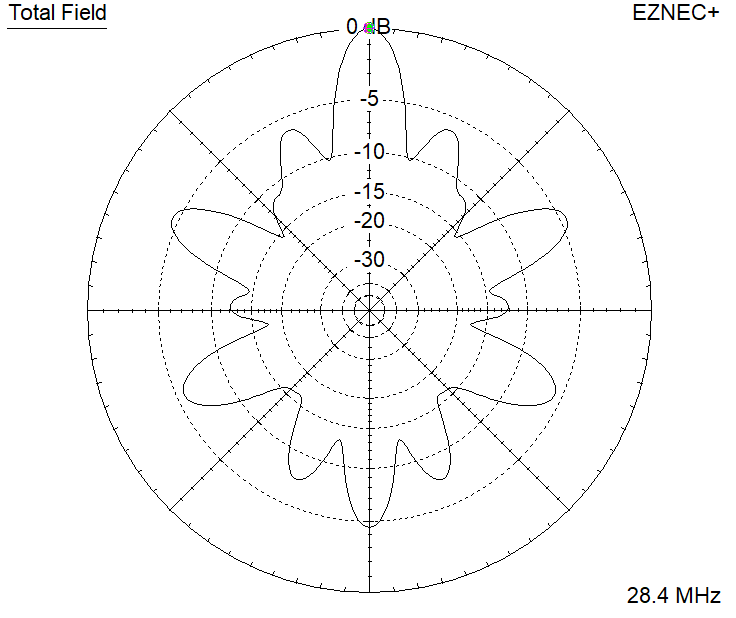
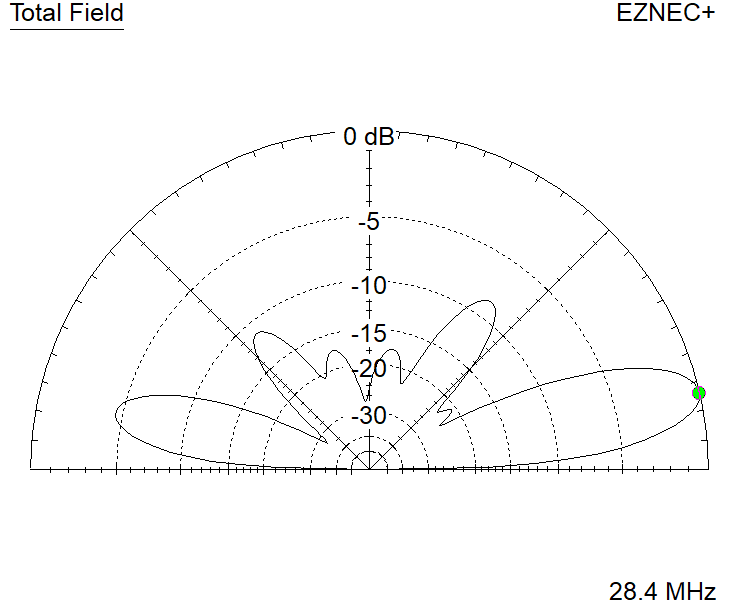
The triangle fed in a corner has a very narrow lobe opposite the feedpoint, with smaller lobes in 5 other directions that are about the same strength as a dipole, along with some higher angle lobes.
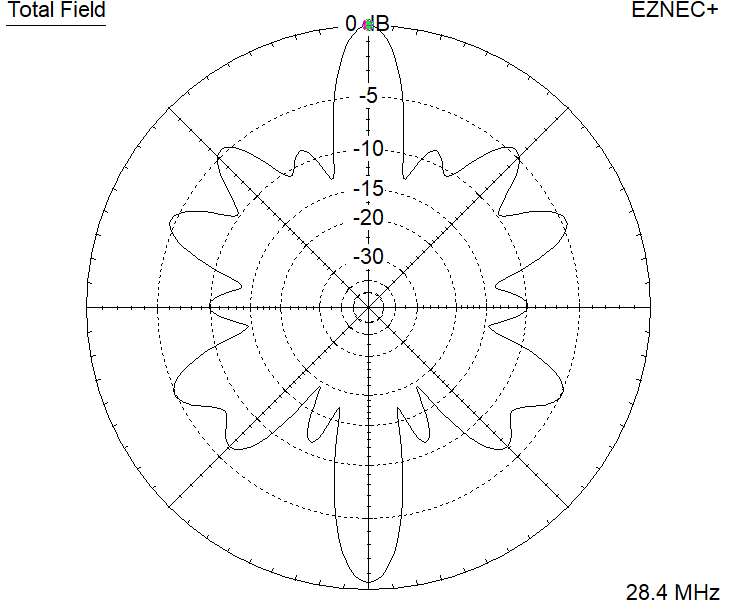
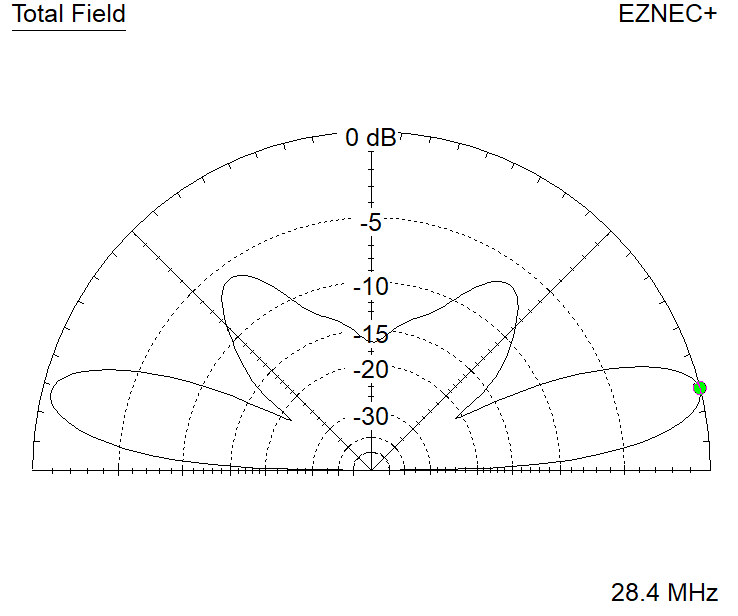
On 10m, the triangle fed in one side has a narrow bidirectional pattern, with a total of 16 identifiable lobes. It would require careful aiming to aim the main lobe at a particular station.
Summary
The 80m loop does make an effective multiband antenna that works reasonably well across all HF bands. On the higher frequencies the radiation pattern breaks up into multiple lobes: it can cover many directions, but with poorer performance in others.
The exact details of the pattern will depend on how accurately the dimensions of the loop can be maintained: this may be an issue in the field where available trees to support it might not be ideally positioned. Generally, maximum radiation is in the direction opposite from the feedpoint, but if this is critical in your application then you may want to model your proposed shape to make sure it points in the desired direction.
These patterns will scale to other bands: for a full wave loop on 160m, the plots at half the listed frequency should apply. Generally speaking, the loop should be dimensioned to be 2 wavelengths on the lowest band where low angle radiation is preferred over high angle radiation. However, the breakup of the pattern into multiple lobes on the higher frequencies needs to be considered.
Vertical angle of radiation is determined to a large extent by height above ground: on the bands where the loop is 2 wavelengths or longer, the tendency of the loop to radiate “off the edges” helps to keep the high angle radiation to a minimum.
BACK TO:
RELATED LINKS
analysis of current distribution on a wire
80m horizontal loop for Field Day
construction of wire loop antennas
EXTERNAL LINKS:
W4RNL: all band use of horizontal loop antennas
W4RNL: horizontally oriented, horizontally polarized large loop antennas